The Acrobatic Bat and Its Sensing Hairs
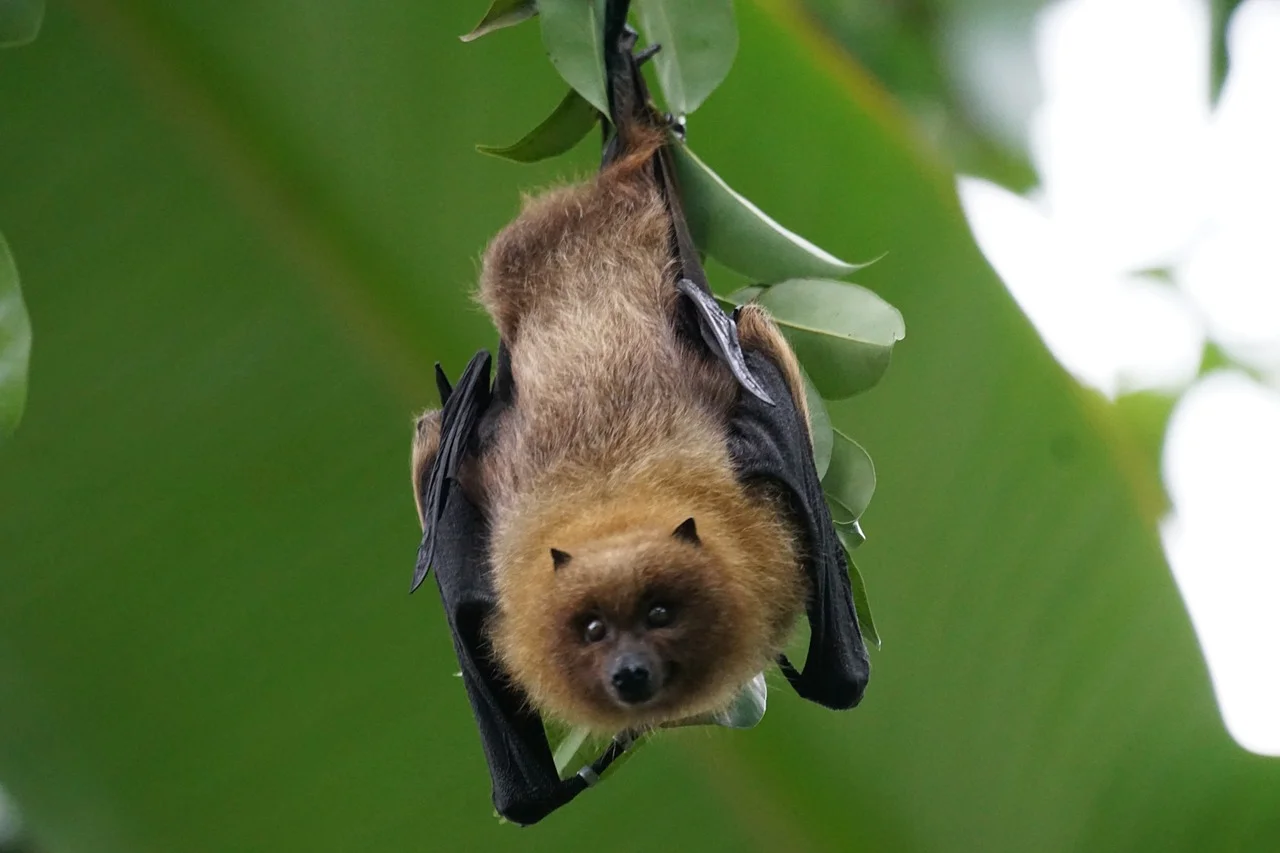
As the sun sinks over the west end of the 400-acre freshwater lake, the 150-foot cottonwood trees slow from leaf-rustle to trunk-sway. The damp air penetrates my nostrils as a sudden shadowy movement catches my eye, and its flutter triggers my hearing. Sinking deeper into the lawn chair sling and craning my neck toward the sky, I observe a baseball-sized figure banking left, taking a 90-degree climb, banking right, and diving. The air wake ruffles my cowlick. Compared to the swallow's daytime dance, this deceivingly clumsy-looking nighttime flight performance displays the telltale signs of a brown bat feeding on mosquitoes. As the only mammal capable of winged flight, the highly skillful bat hovers and tackles 180 degree turns at 200 degrees/second yet uses 20-25% less power than birds. How is this unique mammal capable of the same maneuvers as the U.S. Navy Blue Angels?
Similar to an airplane's flaps that extend and contract to change the lift capacity during take-off and landing, the bat's multi-jointed limbs can adjust the shape of its wings. The bat's infinitely adjustable wings adapt to changing airspeeds and conditions quickly to maintain ideal wing lift. But how can the bat know the required wing shape second-to-second in the near dark?
The bat body has two types of hairs: a longer, softer hair similar to the fur on a short-haired cat, and a second shorter, coarser hair strategically located in rows at the mid-areas and leading edges of the wings. Do you remember flying paper airplanes as a kid? They often flew straight up, stopped mid-air, and crashed straight down to the ground; this mid-air stop is called a stall. When airplanes reach a critical vertical angle, the airflow across its wing becomes turbulent, and the airflow along its wing surface separates (boundary layer separation). This separation causes the lift to reduce and potentially disappear altogether. The location of the bat's shorter hairs is in areas where airflow turbulence and separation begins. The microscopic, single follicle hairs are made of the protein alpha-keratin and have a thick base tapering to a smaller diameter tip. These flexible hairs, which exit from individual cellular domed-structures, bend according to local airflow conditions, activating special sensing cells. The cells' signals inform the bat how to change the shape of its wing for optimal flight performance. This optimal performance allows for the bat's wonderful sky dance.
From the biological knowledge gained from bat hairs, engineers are developing microscopic airflow sensors that could be incorporated into flight control monitoring of micro-air-vehicles, such as drones. Engineers could also mount these mini-sensors on land-based vehicles allowing adaptive aerodynamic systems to improve fuel economy based on vehicle speed. A renewable energy wind turbine blade could use these bat-hair-sensors to adapt the blade shape for maximum efficiency based on wind speed and direction.
What if we reversed the bat-hair-sensor concept moving through the air to the bat-hair sensor remaining stationary as air flowed across it? This airflow energy could be captured and converted for use to advance nano-scale piezoelectric material (microscopic ceramic material that creates an electric current when pressure is applied). Imagine a jacket made of a renewable fleece-like-material creating heat energy from the winter wind. Or a furry bicycle frame that creates energy during fast downhill rides. Nature's flora and fauna evolution over billions of years provide infinite wisdom for sustainable and regenerative concepts the human mind has yet to implement.
So imagine sitting along a water's edge at dusk and let the bat's hair-raising dance tantalize your brain. What other sustainable ideas come to mind?
References:
Dickinson, B.T. (2010) “Hair receptor sensitivity to changes in laminar boundary layer shape.” Bioinspiration & Biomimetics 5 (2010), 016002
Sterbing-D’Angeloa,S, Chadhab,M, Chiuc,C, Falkc,B, Xianc,W, Barceloc,J, Zookd,J and Moss,C (2011). “Bat wing sensors support flight control”. PNAS. Vol. 108 No. 27 p11291–11296
Marshall, Kara L.; Chadha, Mohit; Moss, Cynthia; Lumpkin, Ellen; (2015). “Somatosensory Substrates of Flight Control in Bats.” Cell Reports. 11, 851–858