A Biomimicry Buzz Around Bee Flight and Turbochargers
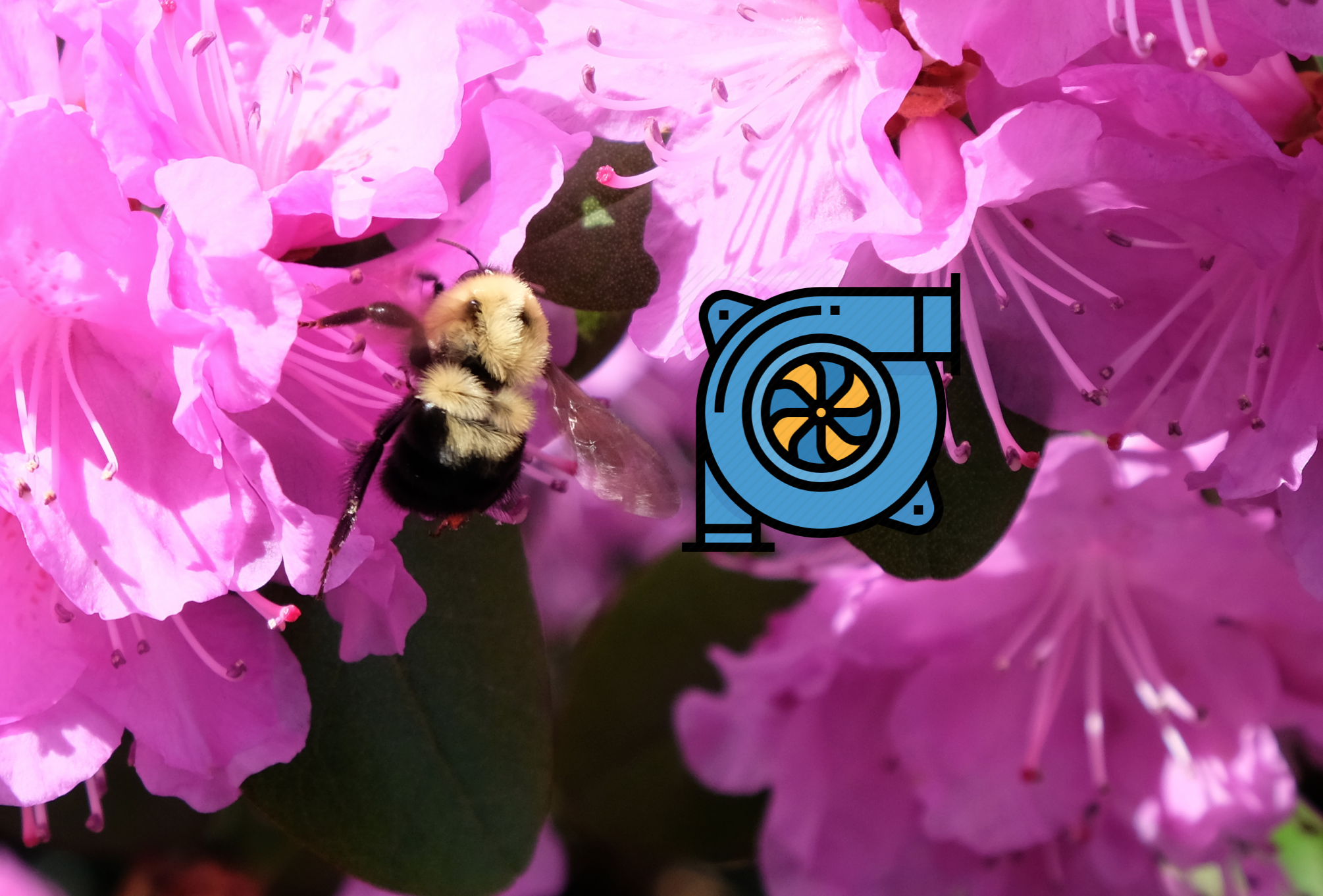
With Michigan lilies and tulips in bloom, the first bumblebee buzzed my head foraging for spring sources of pollen. Somehow the dancing of the bees as they scute from flower-to-flower piques the "enginerd's" mind. How does the bee fly? For 350 million years, insects have evolved to fly differently than birds and bats. Due to a bee's contrasting flight principles, scientists initially argued that they should not fly. With recent advancements in video and computer analysis, the secrets to bee flight are emerging.
The act of hovering (i.e., lift with zero velocity) incorporates a complex series of wing movement patterns. The wing motion consisted of a fore-aft wing stroke similar to how we as humans tread water. The main aerodynamic force created during the bee wing forward stroke is mostly flat; the rearward stroke follows a shallow U-shape pattern. Each part of the stroke: mid-, top, and the bottom, has specific characteristics to create the bee's overall aerodynamic lift.
At mid-stroke, the bee's wing reaches a constant velocity, and steady-state aerodynamic forces develop at the wing's leading edge with a lift-producing vortex similar to how an airplane wing produces lift. Engineers design airplane wings with a 9-degree or less attack angle to prevent turbulent air from detaching from the wing and stalling the lift force. The bee wing angle of attack, however, averages between 41.1 - 50.5 degrees. Because the bee's wing sweeps around its abdomen and not like the airplane wing's fixed position, an additional vortex develops and moves outward along the wing from the abdomen to about 3/4 the length of the wing. This wingspan-wise vortex interacts with the airflow perpendicular to the wing, keeping the air attached to the wing at higher attack angles.
At both the top and bottom stroke, the bee's wing rotates along its span-wise axis to orient the leading edge into the direction of travel. This motion is similar to a human with hands stretched out to the side, rotating their hands from palms up to palms down. During the top stroke, the bee wing changes directions before wing rotation, while at the bottom stroke, the wing rotates first then changes direction. The rotation at stroke reversal creates unsteady lift forces that reduce the time-delay to develop the mid-stroke leading-edge vortex enhancing the steady-state lift. These unsteady forces include rotational, acceleration, and wake capture. The rotational force creates an air circulation around the wing tip circumference and jump-starts the leading-edge vortex for the mid-stroke constant velocity. The acceleration force of the wingtip during rotation creates a linear acceleration force of the wingtip. The wing reversal's wake capture force causes the new leading-edge vortices to interact with the previous trailing edge vortices supplementing the new leading-edge vortex generation.
At the top stroke with both wings closest to each other, the aerodynamic lift phenomenon called "clap and fling" is created. With wings together (clap), the vortices of both wings cancel each other and produce an air jet that enhances thrust. The wing reversal (fling) creates a low-pressure zone that aids in the creation of new vortices.
For a bee to move forward and rearward, the wing's fore-aft stroke plane is tilted forward and backward to transition from hover to linear motion. During low-load hovering, the wing operates within a top-to-bottom stroke window of 90 degrees at about 230 Hz. The bee's indirect flight muscles are tuned for this higher frequency atypical of other insects. To increase linear velocity or the hovering lift force, the wing stroke velocity and distance increase while maintaining 230Hz. This stroke distance increase is biased toward the top stroke. Maintaining frequency with a longer stroke means the wing spends a longer distance creating lift forces at the mid-stroke and at a higher speed where lift force is proportional to the wing tip velocity squared. At a maximum stroke, the bee can fly at 3/4 mph. This increase of stroke technique also increases lift force during hovering, such as when the bee carries more pollen. Because the wing stroke moves toward the top of the stroke, the center of pressure moves up and rearward to offset the pollen load at its feet.
From the perspective of an automotive engineer, the unique procedure in which bee wings develop lift could, via biomimicry, inform the design of climate control blowers and radiator cooling fans. Additionally, the industry-wide increase in turbocharger compressor vanes and turbines or supercharger rotors could achieve unique efficiency enhancements from insect flight knowledge. Even though the bee operates in the air, the lessons learned could inspire unique liquid-based concepts in the realm of coolant and oil pumps. What other bee flight-based concepts come to mind?
References:
Altshuler D, Dickson W, Vance J, Roberts S, Dickinson M. (2005). Short-amplitude high-frequency wing strokes determine aerodynamics of honeybee flight. PNAS. 102(50): 18213-18218. www.pnas.orgcgidoi10.1073pnas.0506590102
Sane, Sanjay P. (2003) The Aerodynamics of Insect Flight. The Journal of Experimental Biology. 206: 4191-4208. https://www.ncbi.nlm.nih.gov/pubmed/14581590
Vance J, Altshuler D, Dickson W, Dickinson M, Roberts S. (2014) Hovering Flight in the Honeybee Apis mellifera: Kinematic Mechanisms for Varying Aerodynamic Forces. Physiological and Biochemical Zoology 87(6):870–881. https://www.jstor.org/stable/10.1086/678955
Wang, Z. Jane. (2005) Dissecting Insect Flight. Annual Review Fluid Mechanics. 37: 183-210. https://www.annualreviews.org/doi/abs/10.1146/annurev.fluid.36.050802.121940